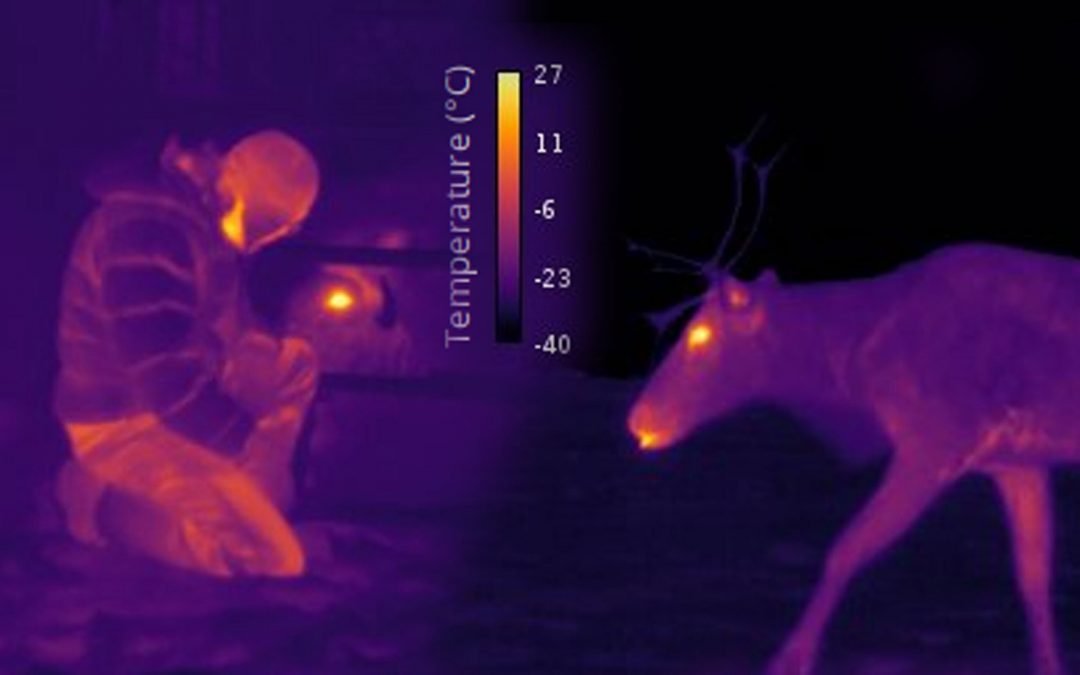
Surviving a Cold Snap
Surviving a Cold Snap
Preface
A few weeks ago, my partner and I had the beautiful fortune to welcome a baby into our family. For me (and my partner), the birth of this baby has meant stepping into the joyous and mystifying waters of parenthood for the very first time. Quite simply, we’re exhilarated. And like most first-time parents, we’re also, perhaps, a touch naive. Despite months of preparative reading and numerous discussions with other early parents, hardly a few hours had passed after his birth when questions began to percolate. How long should feedings last? Is co-sleeping preferable to sleeping separately? And the question most influenced by my career biases: how can we tell if our baby is sufficiently warm, or overheating?


- Extreme cold and extreme heat for many animals can be, and often is, a real threat to their survival, and,
- Facing this threat has paved the way for the evolution of some truly spectacular thermoregulatory strategies and capacities.
Evolution can be a brutal, yet wonderful process.
Thermoregulating in a Cold Snap
In this post, we’ll be taking a look at how certain endotherms at the Yukon Wildlife Preserve are challenged by cold snaps that would undoubtedly prove lethal to an unprepared human. In doing so, we’ll also take a look at how these animals compare to one another in their thermoregulatory strategies and capacities. If you’re like me, I think you’ll find the conclusions intriguing. So let’s put aside the sombre tone and see what findings emerge.
Estimating the Cost of Thermoregulation
Before I begin, I’ll be honest and state that the manner by which I’ve estimated costs of thermoregulation may seem more convoluted than necessary. Actually, in some ways, it probably is more convoluted than necessary. But it does reflect standard practice in the field of thermal biology (for good reason), and it does provide opportunities to paint a fuller picture of what is happening across an animal at the time that an infra-red thermographic picture was captured. With that in mind let’s begin.



Now what about the costs of heat-loss? To estimate this value, a little more calculation will be required. First, you may recall from high-school physics class that heat can be lost in any of three ways: conduction, convection, and radiation. The first method describes the transfer of heat from a solid object to another solid object (think of your lap after being warmed by a cat). By contrast, the other two methods described transfer of heat from a solid to a fluid, and to empty space (think of your hands after warming them by the fire). Estimating heat-loss by each method requires the use of several biophysical theories and equations (nicely summarised by Dominic McCafferty and others), but there are a few common and convenient threads running through them. For example, calculating heat-loss by each method requires knowledge of:
- The temperature differential; that is, how big the temperature difference is between the warm object and the “thing” that it is losing heat to,
- Resistance; that is, how much an object opposes heat flow (this value factors into properties of an object called the “heat transfer coefficient”, or “thermal conductivity”), and,
- Surface area; that is, how much space there is for heat to transfer between a solid object and the “things” it is in contact with.
If we think about it breifly, these values, or parameters, are actually quite intuitive. Big temperature differentials, like those Jake experienced between his body and the air, mean big heat losses. Similarly, low resistance and a large surface area, like those experienced by our large and poorly-insulated moose, also mean big heat losses. For our calculations of heat-loss, we can make strong guesses at resistance and temperature differential values by looking at previous studies on heat emission from biological tissues, and by – you guessed it – using the temperature values that we obtained from our thermal images.

Moose and Muskox at -34°c
To begin, let’s revisit a few candidates from last week: the Moose and Muskox (depicted below). Sadly, we weren’t able to capture any images of the Thinhorn Sheep during the cold snap, so we’ll have to leave that species to our imagination for today.
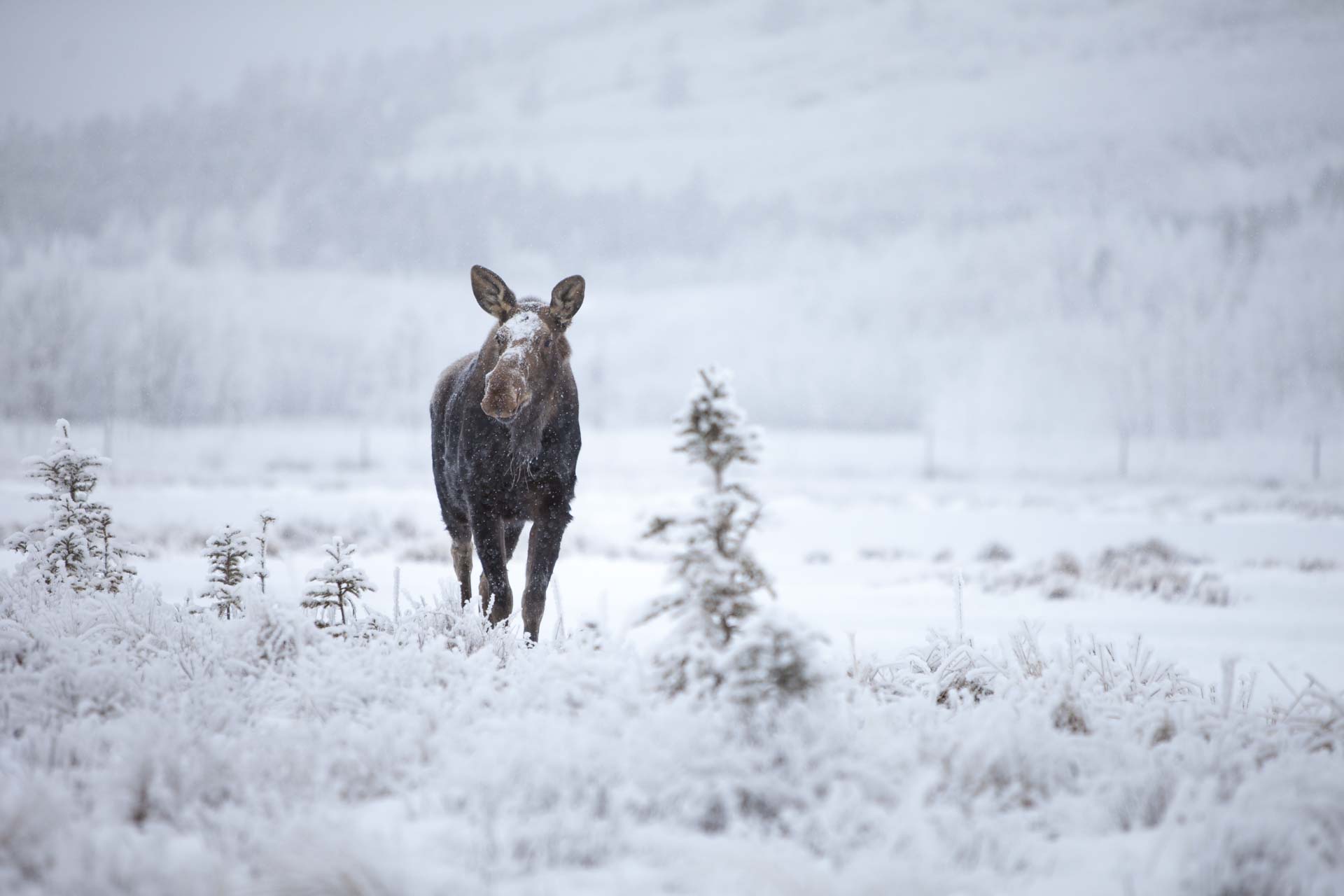
Surface temperatures of muskoxen were only 5°C above ambient temperatures at -30°C, a testament to the substantial insulation provided by their coat…”
Okay, appreciation might be a strong word, but the above quote is probably the closest one can get to appreciation in terse scientific language.
Caribou and Arctic Fox: Cold Weather Specialists
So what about some other Yukon residents? And what about Jake? Next, let’s have a look at few other winter specialists: the Woodland Caribou and Arctic Fox (shown in the images below).
Bison and Mule Deer: Non-specialists?
Finally, to help us contextualize thermoregulatory expenditure in our specialists, I think it will be useful to compare their performance during our cold snap against some local “non-specialists”. (Note that I quote non-specialists here because when compared to an Ocelot or Marsh Widowbird, the below species are truly quite efficient. Nevertheless, the Yukon largely represents the northern end of each species’ range). Below are two infra-red thermographic images of a few conspicuous species meeting this criteria: the Wood Bison (left) and Mule Deer (right).
Comparing Specialists and Non-specialists
Overall, these values do seem to suggest higher expenditure in our non-specialists when compared with our specialists. If we wish to make meaningful conclusions from these values, however, we’ll need to make one important adjustment: scale our estimates of thermoregulatory expenditure against usual daily expenditure (as we discussed in our last last discussion). So far, we’ve quantified the absolute amount of energy each of our observed animals would need to spend to maintain a constant body temperature in the cold. But each of these animals will already be consuming vastly different quantities of stored energy. So by comparing these absolute values among animals, we’re effectively comparing bananas to shoes (or Whitehorse to Destruction Bay); they’re incomparable. To solve this problem, we’ll need to look at relative expenditure toward warming. To follow what I mean here, take a look at the diagram below.

But what about us humans?
From experience, we know that even in full winter clothing, -34°C can be very uncomfortable. And using our imagination, we can also assume that removing our winter gear in such cold weather is a dangerous endeavour. So what does this discomfort and danger mean for our energy expenditure?

- Although eating plenty of cheeseburgers might improve one’s comfort at -34°C, doing so wont keep hypothermia at bay if one remains unclothed. Rather, the extent to which any animal can elevate it’s metabolic rate is limited, regardless of how much food they eat. In humans, that limit seems to fall fairly close to the increase we might have seen in Jake if he were able to maintain a constant body temperature without his jacket. However, that limit appears only to be reached in extreme and long-lasting sporting competitions, such as the Tour de France.
- Adaptations to persist in cold have undoubtedly been helpful for surviving winters in the Yukon, but there is a second side to this coin: a loss of tolerance to warm weather and a bigger battle to face when coping with climate change.
Our Muskox and Arctic Fox might have an interesting future ahead of them.

Joshua Robertson
Behavioural and Physiological Ecologist
Joshua is a behavioural and physiological ecologist currently living on Cape Breton Island, Nova Scotia. During his PhD at Trent University, Joshua sought to understand how small birds can cope with the high costs of body temperature regulation when challenged with other environmental stressors (such as human and predator exposure). He is currently extending the research to better understand energy management strategies in warm-blood animals.